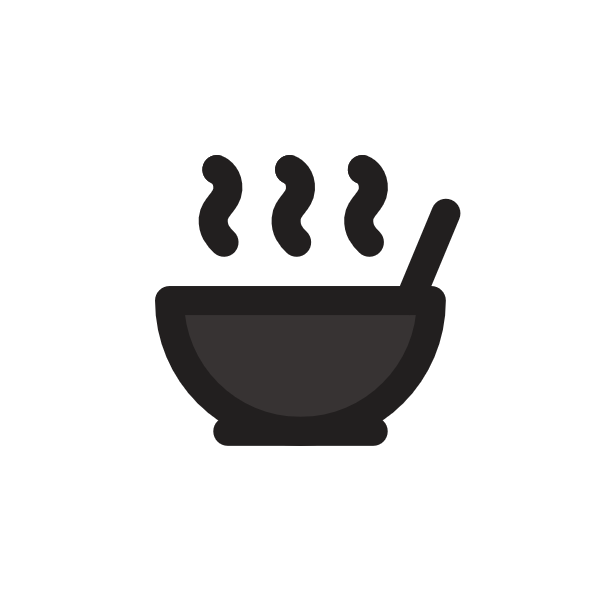
Cocojunk
🚀 Dive deep with CocoJunk – your destination for detailed, well-researched articles across science, technology, culture, and more. Explore knowledge that matters, explained in plain English.
Bubble memory
Read the original article here.
Bubble Memory: A Lost Pioneer in Non-Volatile Storage
In the ever-evolving world of computer technology, some innovations emerge with great promise, only to be eclipsed by newer, more efficient solutions. Bubble memory stands as a fascinating example of such a technology – a non-volatile memory system developed in the 1970s that offered a unique combination of features, positioning it as a potential "universal memory." Despite its initial allure and niche applications, bubble memory ultimately faded into obscurity with the rise of semiconductor memory and hard disk improvements. This article delves into the history, technology, and reasons behind the rise and fall of bubble memory, highlighting its place as a "lost innovation" that was undeniably ahead of its time.
What is Bubble Memory?
At its core, bubble memory is a type of non-volatile memory. This means that, unlike RAM (Random Access Memory), bubble memory retains data even when the power is turned off. This characteristic was a significant advantage in an era where data persistence was often reliant on more cumbersome technologies like magnetic tapes and disks.
Non-Volatile Memory: Computer memory that can retain stored information even after power is removed. Examples include ROM, flash memory, and historically, bubble memory and magnetic core memory.
Bubble memory utilizes a thin film of magnetic material to store data. Within this film, tiny magnetized regions, known as bubbles or domains, are created and manipulated to represent bits of data. Each bubble, or its absence, corresponds to a binary digit – 1 or 0.
Magnetic Domains (Bubbles): Microscopic regions within a magnetic material where the magnetization is uniform. In bubble memory, these domains are cylindrical and are used to store data.
Think of it like this: imagine a grid of tiny, controllable magnets on a film. Each magnet can be either "on" (bubble present - representing '1') or "off" (bubble absent - representing '0'). These bubbles are not static; they can be moved along defined tracks within the material using an external magnetic field.
The operation of bubble memory shares similarities with delay-line memory systems. In delay-line memory, data is stored as a sequence of signals that propagate through a medium (like a mercury tube or acoustic delay line) and are recirculated to be accessed again. Similarly, in bubble memory, the bubbles are moved along tracks, and by recirculating them, the memory can retain the data.
History of Bubble Memory
Precursors: Laying the Groundwork
The story of bubble memory is intrinsically linked to the pioneering work of Andrew Bobeck. His prior experience with magnetic memory technologies in the 1960s at Bell Labs was crucial in shaping the development of bubble memory. Two projects, in particular, played a significant role:
Magnetic-Core Memory with Transistor Controller: Bobeck worked on developing the first magnetic-core memory system controlled by transistors. Magnetic-core memory was a dominant form of RAM before semiconductor memory, utilizing tiny ferrite rings (cores) magnetized in different directions to store bits. Transistors offered a more efficient and reliable way to control the reading and writing of data in these cores.
Twistor Memory: Twistor memory was an attempt to improve upon core memory by automating the manufacturing process. Instead of individual ferrite cores, twistor used a magnetic tape wrapped around wires. The idea was that twistor could be mass-produced more easily than core memory, which was largely assembled manually. AT&T envisioned twistor as a cost-effective memory solution.
Twistor Memory: A type of magnetic memory developed by Bell Labs. It used magnetic tape wrapped around wires to store data. While designed for automated assembly, it was ultimately overtaken by DRAM.
However, fate intervened in the form of DRAM (Dynamic Random-Access Memory). DRAM emerged in the early 1970s and quickly surpassed both core memory and twistor in terms of speed, density, and cost-effectiveness for random-access applications. Twistor found limited use, primarily within AT&T's own systems.
Interestingly, during the development of twistor, a key observation was made: passing current through the wires within the magnetic tape could cause the magnetic fields (and thus the stored bits) to move along the tape in the direction of the current. This hinted at the possibility of controlling the movement of magnetic data, a concept that would later be central to bubble memory. This early observation, while not directly leading to twistor's success, planted a seed for the future of magnetic domain manipulation.
Development: From Domains to Bubbles
In 1967, Bobeck joined a team at Bell Labs specifically to work on improving twistor memory. The density of twistor memory was limited by the size and spacing of the wires. Conventional magnetic materials, like those used in tape, allowed magnetic signals to be placed and moved in any direction.
A crucial breakthrough came with the work of Paul Charles Michaelis, who discovered that using permalloy (a nickel-iron magnetic alloy) thin films enabled the movement of magnetic signals in orthogonal (perpendicular) directions. This discovery, patented and presented in 1967, was a significant step towards controlled magnetic domain movement. However, this initial method used anisotropic magnetic films, meaning their magnetic properties differed depending on the direction of magnetization. This made movement complex and direction-dependent.
The need for a more uniform magnetic medium led Bobeck to experiment with orthoferrite. While working with orthoferrite, he observed a fascinating phenomenon. When a magnetic domain was written onto the orthoferrite and then a magnetic field was applied across the entire material, the domain would shrink into a tiny, stable circle – a bubble. These bubbles were significantly smaller than the domains in materials like magnetic tape, suggesting the potential for much higher data densities.
This discovery, combined with subsequent research at Bell Labs, led to five key advancements that formed the foundation of bubble memory:
Controlled 2D Motion in Permalloy Films: Michaelis's work demonstrating the movement of magnetic domains in two dimensions within permalloy films.
Application of Orthorferrites: Recognizing the potential of orthoferrite materials for bubble formation.
Discovery of Stable Cylindrical Domains (Bubbles): Bobeck's observation of the stable bubble formation in orthoferrite.
Invention of Field Access Mode of Operation: Bobeck's crucial invention of using an external rotating magnetic field to move the bubbles, a more practical approach than directly driving each bubble individually.
Discovery of Growth-Induced Uniaxial Anisotropy in Garnets and Garnet Materials: Recognizing that garnets, a class of silicate minerals, specifically garnet films, possessed the ideal magnetic properties for bubble memory and could be practically manufactured. These materials exhibited isotropic magnetic properties, making bubble manipulation more predictable and efficient.
Garnets: A group of silicate minerals with specific magnetic properties that proved ideal for bubble memory. Garnet films, grown epitaxially on substrates, became the primary material for bubble memory chips.
The development of bubble memory was not a singular invention, but rather a culmination of these discoveries and the collaborative efforts of a large team at Bell Labs. The IEEE recognized the significance of this work by awarding H.E.D. Scovil, P.C. Michaelis, and Andrew Bobeck the Morris N. Liebmann Memorial Award in 1974 for "the concept and development of single-walled magnetic domains (magnetic bubbles), and for recognition of their importance to memory technology."
The challenge then shifted to controlling the movement of these bubbles within a 2D plane. While orthoferrite allowed bubble formation, it didn't inherently guide their movement in a controlled manner. The solution was ingenious: propagation elements.
Propagation Elements: Tiny patterns of magnetic material, typically permalloy, imprinted onto the garnet film surface. These elements, often shaped like T-bars or chevrons, acted as guides for the bubbles. When a rotating magnetic field was applied, these elements would become magnetized, attracting the bubbles and guiding them along predefined paths. By precisely controlling the rotating magnetic field, the bubbles could be moved, stored in loops, and retrieved for reading.
Commercialization: Promise and Decline
By the early 1970s, Bobeck's team had created bubble memory chips capable of storing 4,096 bits on a 1 cm² chip, matching the density of then-standard core memory. This sparked immense excitement within the electronics industry. Bubble memory was envisioned not just as a replacement for core memory, but potentially for tapes and disks as well – a "universal memory" solution.
Early experimental devices using bubble memory emerged from Bell Labs in 1974. By the mid-1970s, major electronics companies like Texas Instruments, Intel, Rockwell, National Semiconductor, and Plessey, all invested heavily in bubble memory research and development.
Texas Instruments launched the first commercial product with bubble memory in 1977 and followed up with the first commercially available bubble memory chip, the TIB 0103 (92 kilobits). Intel entered the market in 1979 with a 1-megabit bubble memory chip, the 7110. By the early 1980s, 4-megabit chips were available, and even 16-megabit versions were under development.
However, the initial optimism surrounding bubble memory was short-lived. Two key factors led to its rapid decline:
- Advancements in DRAM: Semiconductor memory technology, particularly DRAM, continued to improve dramatically in speed and density, while also becoming cheaper to manufacture. DRAM quickly outpaced bubble memory in performance for random-access applications.
- Hard Drive Revolution: Hard disk drive technology also experienced significant breakthroughs in capacity and cost during the early 1980s. Hard drives became the dominant solution for mass storage, offering much higher capacity at a lower price point than bubble memory.
By 1981, many major companies, including Rockwell, National Semiconductor, Texas Instruments, and Plessey, had shut down their bubble memory operations. A smaller group, dubbed the "big five" (Intel, Motorola, Hitachi, SAGEM, and Fujitsu), continued to pursue "second-generation bubble" technology, but the writing was on the wall.
Bubble memory found a niche in applications where its non-moving nature was a significant advantage. Its robustness against mechanical shock and vibrations, and its low maintenance requirements, made it suitable for:
- Harsh Environments: Industrial control systems, military applications, and portable equipment operating in demanding conditions where disk drives were unreliable.
- Arcade Games: Konami's Bubble System arcade platform (1984) utilized interchangeable bubble memory cartridges for game storage, offering faster loading times than floppy disks of the era.
- Early Laptops: Sharp (PC 5000 series, 1983) and GRiD Systems Corporation incorporated bubble memory in their early portable computers.
- Specialized Instruments: Nicolet and HP used bubble memory in oscilloscopes and signal analyzers for waveform storage due to its reliability and non-volatility.
- Telecommunications: TIE communication utilized bubble memory in early digital phone systems to improve reliability and provide non-volatile memory for system configurations.
- Visual Effects Systems: Quantel Mirage DVM8000/1 VFX system employed bubble memory.
However, even these niche applications were eventually overtaken by the emergence of flash storage. Flash memory offered a compelling combination of non-volatility, solid-state reliability, higher density, faster access speeds, and lower costs compared to bubble memory. By the late 1980s, bubble memory had largely disappeared from the market.
Inside Bubble Memory: Technology and Operation
A bubble memory device is a complex assembly, carefully engineered to create and manipulate magnetic bubbles for data storage. Here's a breakdown of its key components and operation:
Components:
- Case and Magnetic Shielding: The entire assembly is housed in a case that often acts as a magnetic shield. This shield is crucial for isolating the sensitive magnetic bubbles from external magnetic interference and also provides a magnetic return path for the internal magnets.
- PCB (Printed Circuit Board): The electronic components and bubble memory chips are mounted on a PCB.
- Bubble Memory Chips: These are the heart of the system. They are typically translucent and contain the garnet film where the magnetic bubbles are stored.
- Windings (Coils): Two sets of windings, usually made of copper wire, surround the bubble memory chips. These windings are oriented perpendicularly (e.g., along the X and Z axes) and are driven with alternating currents to generate a rotating magnetic field.
- Permanent Magnets: Two powerful permanent magnets, positioned above and below the windings and chips, create a static (DC) bias magnetic field. This bias field is essential for maintaining the stability of the bubbles and ensuring non-volatility. Without these magnets, the bubbles would collapse, and data would be lost.
- Propagation Elements (Chevrons, T-bars): Microscopic patterns of ferromagnetic material (like permalloy) are fabricated on the surface of the garnet film. These propagation elements guide the movement of the bubbles under the influence of the rotating magnetic field. Asymmetrical chevrons became a common design for these elements.
- Boot Loop: A special, separate loop on the chip used to store information about defective storage loops on the memory chip. This is read at startup to manage manufacturing imperfections.
Operation: Reading, Writing, and Storing Data
Bubble Generation (Writing Data):
- A seed bubble is continuously present and circulated in a dedicated area.
- To write a '1' (create a bubble), a hairpin-shaped conductor (made of material like aluminum-copper alloy) is briefly energized with a strong current pulse. This pulse creates a localized magnetic field that opposes the bias field, causing the seed bubble to be "cut" or split. One part of the split bubble becomes a new data bubble.
- The newly generated bubble is then guided into an input track and subsequently into a storage loop.
- To write a '0', no current pulse is applied, and no new bubble is generated in that bit position.
Data Storage (Retention):
- Storage Loops: Bubbles are stored in rows of storage loops. These loops are formed by the propagation elements and allow bubbles (and empty spaces representing '0's) to circulate continuously under the influence of the rotating magnetic field.
- Non-Volatility: The permanent magnets maintain the bias field, ensuring that bubbles remain stable within the storage loops even when power is removed. This provides non-volatile data retention.
Bubble Detection (Reading Data):
- Replication: To read a bubble, it is first "replicated." This involves stretching the bubble by moving it to a larger propagation element.
- Splitting: The stretched bubble is then passed under another hairpin-shaped conductor, which is pulsed with a current to split the bubble in two. One copy remains circulating in the storage loop, preserving the data. The other copy is directed towards the detector.
- Detector (Magnetoresistive Bridge): The copy bubble is moved to a magnetoresistive bridge detector. This detector is typically composed of a column of interconnected permalloy chevrons. As the bubble passes under the detector, it slightly changes the electrical resistance of the permalloy material.
- Signal Amplification: A constant current is passed through the detector. The change in resistance due to the bubble's presence causes a small voltage change (in millivolts). This voltage signal is amplified and interpreted as a '1'. The absence of a voltage change is interpreted as a '0'.
- Bubble Destruction: After detection, the copy bubble is guided to a "guard rail" to be destroyed, preventing it from interfering with subsequent operations.
Rotating Magnetic Field: The rotating magnetic field, generated by the windings, is the driving force behind bubble movement. This field, typically rotating at 100-200 kHz, causes the propagation elements to become sequentially magnetized, effectively "pushing" or "attracting" the bubbles along the defined paths.
Materials:
The choice of materials in bubble memory is critical to its operation and performance.
- Gadolinium Gallium Garnet (GGG): Used as the substrate for bubble memory chips. GGG is non-magnetic and provides an excellent crystalline base for the epitaxial growth of magnetic garnet films.
- Garnet Films (Bubble Host/Bubble Layer): The active magnetic layer where bubbles are formed and stored. These films are typically single-crystal substituted yttrium iron garnet containing gadolinium or other elements. They are grown epitaxially on the GGG substrate using liquid-phase epitaxy with lead oxide flux in a platinum crucible. Ion implantation is often used to fine-tune the magnetic properties of the garnet film.
- Permalloy (Nickel-Iron Alloy): Primarily used for propagation elements (chevrons, T-bars) and the magnetoresistive detector. Permalloy is chosen for its ferromagnetic properties, ease of fabrication, and sensitivity to magnetic fields.
- Aluminum-Copper Alloy: Used for the hairpin-shaped conductors for bubble generation and splitting due to its conductivity and ease of microfabrication.
Advantages and Disadvantages of Bubble Memory
Advantages:
- Non-Volatility: Data retention even without power, a key advantage over RAM in its time.
- Solid-State Reliability: No moving mechanical parts, leading to high reliability and robustness, especially in harsh environments, compared to disk drives.
- High Density Potential: Theoretically capable of achieving high storage densities compared to magnetic tape and early disk drives.
- Shock and Vibration Resistance: Immune to mechanical shocks and vibrations due to the absence of moving parts, making it suitable for portable and mobile applications.
- Low Maintenance: Solid-state nature reduced maintenance requirements.
Disadvantages:
- Slow Access Speeds: Access times were significantly slower than semiconductor RAM. Data access was serial, requiring bubbles to be physically moved to the detector.
- Lower Storage Capacity Compared to Hard Drives: While denser than core memory, bubble memory capacity was quickly outpaced by the rapid advancements in hard disk drive technology.
- Higher Cost Compared to DRAM and Hard Drives: Manufacturing costs were higher than both DRAM and hard drives, making it less competitive in mainstream applications.
- Temperature Sensitivity: Bubble memory performance could be affected by temperature variations and sometimes required heating for optimal operation, as seen in the Konami Bubble System.
- Complex Manufacturing: Fabrication of bubble memory chips was a complex and expensive process.
- Power Consumption: While non-volatile, operation required power for the rotating magnetic field and read/write operations, which was not insignificant compared to static ROM.
Why Bubble Memory Was Lost: The Rise of Alternatives
Despite its innovative nature and niche successes, bubble memory ultimately failed to achieve widespread adoption and was eclipsed by other memory technologies. The primary reasons for its demise are:
- Relentless Progress in Semiconductor Memory (DRAM): DRAM technology experienced continuous and rapid advancements in speed, density, and cost. DRAM quickly became the dominant choice for main memory due to its superior performance for random-access operations.
- Hard Drive Capacity and Cost Breakthroughs: Hard disk drive technology underwent a revolution in the early 1980s, achieving massive increases in storage capacity while significantly reducing costs. Hard drives became the undisputed leader in mass storage, offering far more storage for less money than bubble memory.
- Emergence of Flash Memory: Flash memory, developed in the late 1980s and early 1990s, provided a compelling alternative that combined non-volatility with solid-state reliability, high density, and eventually, competitive speed and cost. Flash memory effectively addressed the niche applications where bubble memory had found some success and ultimately replaced it entirely.
In essence, bubble memory was caught in a technological squeeze. It was too slow and expensive to compete with DRAM for main memory, and it lacked the capacity and cost-effectiveness to compete with hard drives for mass storage. Flash memory then emerged to fill the non-volatile solid-state niche more effectively.
Legacy and Modern Relevance
While bubble memory is no longer commercially viable, its development and the underlying principles of manipulating magnetic domains have contributed to advancements in other areas. IBM's racetrack memory, developed in the 2000s, can be seen as a spiritual successor to bubble memory. Racetrack memory uses nanowires to store magnetic domains and move them along the wire, offering high density and non-volatility. It shares a conceptual link with bubble memory's controlled domain movement and also has a resemblance to the serial nature of twistor memory, highlighting a lineage of magnetic domain manipulation technologies.
Furthermore, the research and development efforts in materials science, microfabrication, and magnetic domain physics spurred by bubble memory contributed to the broader advancement of these fields, indirectly benefiting other technologies.
Bubble memory, though ultimately a "lost" technology, represents a fascinating chapter in the history of computer innovation. It was a bold attempt to create a universal memory solution, and while it didn't achieve its grand ambitions, it served as a valuable stepping stone in the ongoing quest for better and more efficient data storage solutions. Its story reminds us that technological progress is not always linear and that even technologies that fade from the mainstream can leave a lasting legacy and inspire future innovations.
See also
- Gadolinium gallium garnet
References
[Original references from the Wikipedia article would be listed here if provided in the source]
External links
- Great Microprocessors of the Past and Present. Appendix F: Memory Types
- The Arcade Flyer Archive: Konami Bubble System Flyer
- Bubbles: the better memory
- Whatever Happened to Bubble Memory?
- Magnetic Bubble Memories - Web site by George S. Almasi
- Novel Non-magnetic Bubble Memory
- Structure of a bubble memory
- An exploded view and photo of a dissasembled bubble memory, showing PCBs with memory bubble chips
- A file operating system ported to a modern bubble board